Senior Distance Running Essentials Series
Chapter #4: Biomechanics: Fundamentals of Movement (May 2024)
Senior Distance Running Essentials is a sequential series and hopefully you have had a chance to read the first three chapters.
The topic here is biomechanics. The word root “bio” means life, or living, while “mechanics” refers to a machine, instrument, or tool. Therefore, the term “biomechanics” literally refers to the intersection of life and machine—the human body is the ultimate living machine!
The movement of our bodies is caused by forces generated by and to our bodies during activity. In chapter #1, we watched the late elite senior runner Ed Whitlock and considered his form as an example of the working of the kinetic chain. Here’s that link: https://www.youtube.com/watch?v=WaXiBFow9Es. Everything moves in sync and don’t we wish our running looked as smooth and effortless as was Ed’s! Yet, the principles are the same for all of us.
Biomechanics is essential to running. Thus, there are two chapters on the topic. Biomechanics helps us understand how our skeleton, joints, muscles, and tendons work together. We’ll cover basic biomechanical principles in this chapter. The next chapter will examine the biomechanics of the lower extremity, our running chassis.
Joints are of primary importance in our bodily movement. They are the pivot point between bones. Without joints we couldn’t move! Thus, healthy joints are essential for continued running as we age.
Causes of runner attrition
I am not aware of data that reliably quantifies the various reasons why seniors stop running. But we know there is an overall attrition rate of above 60% by age 60. Based on those I know, I suspect much of the attrition is due to failing joints, sometimes from osteoarthritis. Some have had joint replacements and while improvement in these procedures is impressive, replacements still do not typically rival original equipment, and rarely lead to a full comeback.
Lower rate of osteoarthritis in runners
As noted in Chapter 2, a 2017 study found recreational runners incurred hip and knee osteoarthritis at one-third the rate of the nonrunning population. One possible, if not likely, reason is the protective application of Woolf’s Law, which holds that bone is generated, rather than degenerated, in areas of stress.
Synovial joints
The joints we focus on in running are mostly synovial joints in the hip, knee, and ankle. In synovial joints, bones come together with the ends covered by smooth cartilage terminating in a fluid-filled cavity, or capsule, that provides joint lubrication, enabling bones to glide.
Let’s consider an example of the knee joint provided by Elsa Chinburg, a student in the doctor of physical therapy program at UVM and world-class triathlete.
Elsa suggests placing our right fist in the palm of the left hand, allowing the left fingers to relax around the knuckles of the right hand. She sees this as a rough approximation of what our upper and lower leg bones look like when they come together at the knee joint. Elsa notes by spinning and pivoting the right fist, keeping the two hands in contact with each other, this simulates the motion at our knee joint when running. She emphasizes that these two bones must have as little friction as possible so movement can be smooth and seamless.
Here’ a view of the anatomy of the knee. If the biomechanics in the knee are working well, we probably don’t think about it. But if the cartilage is worn – we think a lot about it.
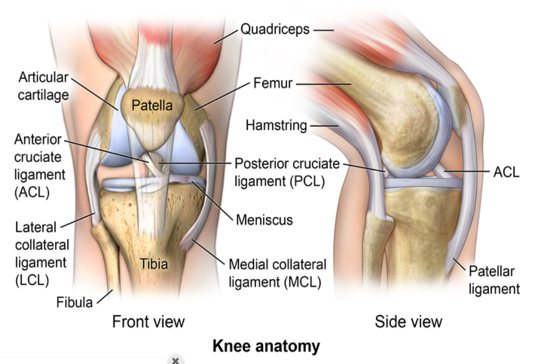
Now let’s consider two fundamental aspects of movement.
First, muscles are what enable movement around a joint. They can only pull, not push. This action is called a contraction, of which there are three types: concentric (e.g. a bicep curl); eccentric (e.g., a reverse curl); or isometric (e.g. push against a wall.) We’ll explore later in the series how we use all three types of contractions when running.
Second, bones function as levers, carrying out the movement initiated by muscles. Bones accomplish this movement via muscles being attached to bones, usually by a tendon.
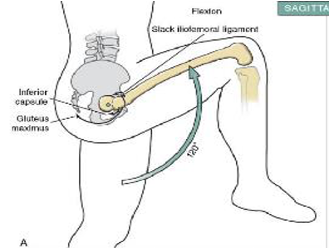
The attachment at one end of the muscle is called the origin, which is generally proximal — meaning toward the trunk — and is fairly stationary during movement; the attachment at the other end is called the insertion, which is typically distal — meaning away from the trunk — and usually moves the attached bone during movement. It is in this section of the tendon between bones and muscles where we often experience pain from tears or strain from repetitive motion.
In the above diagram, attachments at the hip hold the proximal end of the femur in place while attachments near the distal part of the femur allow us to move our leg. In the next chapter we’ll come back to this and look more closely at the origin and insertion of the primary muscles in the lower extremity.
Running is about forward movement. The technical term for this is “linear translation” — we are moving our bodies in a straight path from the start line to the finish. But unlike an arrow being shot from a bow, we need to bring all the parts of our body along with us. We’re not stick figures and some of our parts move up-and-down, side-to-side, and others twist, allowing for a rhythm and balance in our gait.
Cardinal Planes
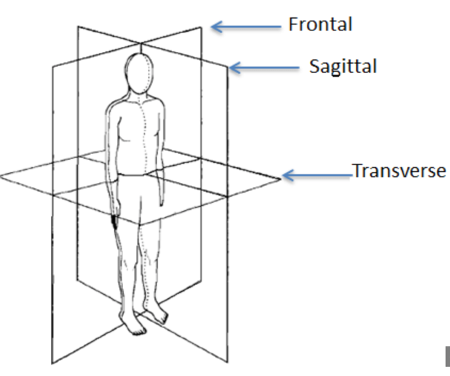
To describe and then measure these elements of movement we use three cardinal planes of motion: sagittal, frontal, and transverse (also called horizontal or rotational) as shown here.
Cardinal in this context means central and each of the three planes divide the body in half from a different perspective.
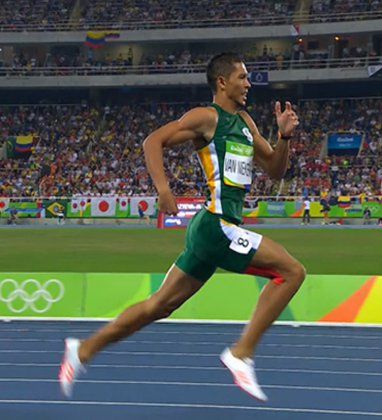
Most running movement is observed in the sagittal plane where we see forward and up-and-down movement. We can observe this from the side when looking at the gait cycle. There is also a small amount of side-to-side frontal plane movement at the hip and a slight twisting, rotational movement also at the hip, as the gait proceeds. When you watch an accomplished runner, such as world marathon record holder Eliud Kipchoge, this fluid motion is apparent. While we may not look quite like Kipchoge, we can work toward having an efficient stride with biomechanics aimed at moving us forward with the least amount of effort. Plyometrics, exhibited later in the series, can help with this.
Newton’s Laws of Motion
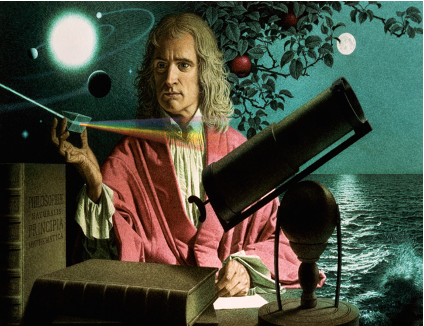
When considering the principles of Biomechanics, there may be no better place to start than Sir Isaac Newton’s three laws of motion. These have been around since 1687 and have thus stood the test of time. While we don’t hear of Newton as a runner, in formulating his laws of how and why a body moves, it’s not a stretch to think of him testing out these laws on the local roads! In any event, we will briefly consider each law and may find occasion to refer to Newton’s Laws during the series.
Law of Inertia
The first is the Law of Inertia that says there can only be movement or a change in movement if a force is applied to a body. We’re standing at the start line, the gone goes off, we put our foot forward — apply force with our leg muscles — and off we go!
Law of Acceleration
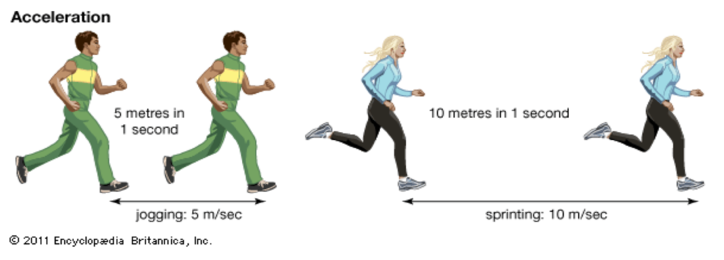
The second law is the Law of Acceleration. It says more force is needed to pick up speed. This is about the rate of change in movement when a force is applied. Thus, we engage more muscle fibers, push the pedal down closer to the metal, and move forward faster! This picture shows two runners, but it could be the same person going from jogging to racing.
Law of Action-Reaction
The third law is the Law of Action-Reaction, or sometimes just called Reaction. This law says for every force applied an equal and opposite force is returned. This comes into play when the force with which we hit a running surface, typically 2 to 3 times our body weight, comes back at us as a ground reaction force.
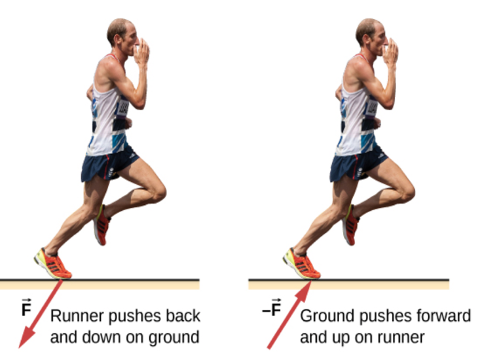
This may be a bit hard to fathom, but from a practical standpoint if we are light on our feet and run on a softer surface, both the impact and this reactive force are reduced, saving our joints from excessive loads. When we plant our foot on the ground, that impact, or ground reaction force, travels up the kinetic chain from our point of impact to other joints (i.e., the knee, hip, and spine) in the body.
This last point reminds me of something I counsel against – running on concrete sidewalks. Take a hammer and hit the sidewalk — it will literallybounce back at you! Then do the same on asphalt – some bounce but not as much. Then for good measure, pound on a dirt path – no bounce!
Surface Matters
The point is surface matters! In addition to hardness, the sectional nature of concrete sidewalks changes the angle of torque coming back as a ground reaction force.
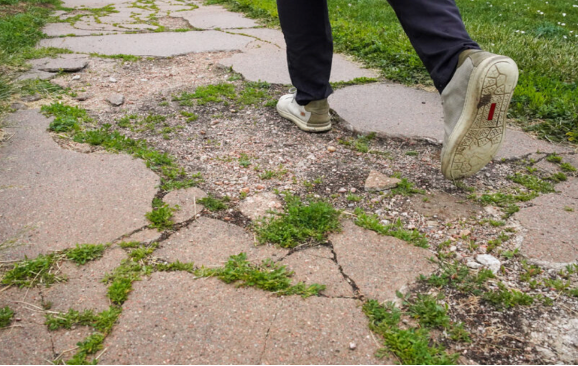
And unless a sidewalk has been recently replaced there can be numerous lips and cracks to catch and trip on. There will be times, especially in winter, when part of our runs on sidewalks cannot be avoided. But many city streets now have bike lanes and we runners have a right to use them, facing traffic of course! Yes, watch the eyes of drivers as cars approach and be ready to jump onto the curb if someone isn’t paying attention or chooses to be downright aggressive. But for the reasons noted, I suggest doing what you can to stay off sidewalks.
A “running conversation” between our body parts
In Chapter #1 we noted there are 206 bones, 360 joints, and 640 muscles. Many of the largest bones and muscles are in the lower extremity. These moving parts need to “talk” to each other, meaning what’s going on in one part affects what happens elsewhere. For example, if we’re running and step sideways on a pothole, a bunch of things happen simultaneously to keep us from injury or at least minimizing injury. First, there is an immediate shift of weight away from the twisting ankle as specialized sensory receptors called proprioceptors help reestablish balance. Then, various muscles engage as limbs establish a new equilibrium. Finally, we regain our balance, hopefully having avoided falling or spraining an ankle, and return to our normal running pace. We don’t have time to think about this – it just happens!
The inside view of movement
What we’ve considered above is the big picture of movement: muscles move the bones around our joints and off we go! But the intricate microscopic processes taking place that allow for that are both precise and complicated. We will take brief look at what is called the “sliding filament theory.”
Our skeletal muscles are composed of bundles of individual muscle fibers. These fibers are composed of myofibril (“myo” means muscle) myosin and actin protein filaments. The sequence below shows how these filaments slide over each other resulting in a “power stroke” that initiates movement. A lot is going on here and a whole chapter could be dedicated to this. If you are curious, Chat GPT does a great job of describing this process.
In any event, with muscle about 75% water and 20% protein, roughly 80% of non-water muscle structure is protein, emphasizing the importance of protein in our diet. We will look at this in the nutrition chapter.
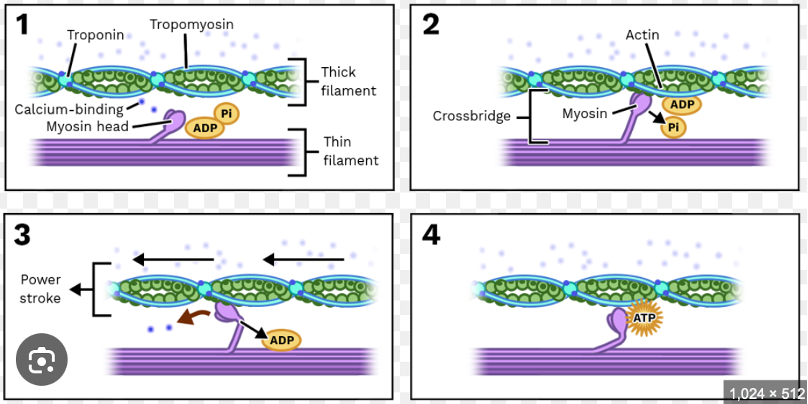
Changes in muscle with age
Since muscles are the drivers of movement, changes in muscle with age affect our ability to move quickly and repeatedly. As noted in Chapter #3, there are cellular changes that affect muscular function. Studies show an average person loses 10% of peak strength each decade after 60, with that loss accelerating after age 75.
What is going on is called sarcopenia, which is a loss not only in the number and size of muscle cells but also involves an infiltration of connective tissue and fat within muscles. This is sad but true! Yes, we can address this loss, in part, by doing maximal strength training. We will look at that in later chapters.
With this introduction, the next episode will go into more specifics. Thanks for reading and please forward a link to others you think might be interested in subscribing to the series.
I hope your running is going well and you are finding this series useful in your training.