Senior Distance Running Essentials Series
Chapter 6: Our Cardiorespiratory System (May 2024)
Welcome to Senior Distance Running Essentials Chapter #6.
“I’d do that in a heartbeat”
Recall the adage: “I’d do that in a heartbeat.” It means we’d do something without any delay or hesitation. It’s fitting that saying is attributed to our heart. If there is any delay or hesitation, we’ve got problems! Our hearts are always on. Even at a runner’s low pulse rate of 50, during an 80-year lifetime the heart beats over two trillion times. Talk about a workhorse!
A partnership — the cardiorespiratory system
But our heart and cardiovascular system could not function without its partner — the respiratory system, which also has to be working nonstop. Like the cardiovascular system, the respiratory system is composed of complex, intricate parts. It warms, humidifies, and filters the air we breathe. And via the lungs delivers oxygen to our body and removes carbon dioxide from it. These two systems operate in a continuous, interrelated way and are accordingly sometimes referred to together as the cardiorespiratory system. I call this the human highway since so much of what is vital to our function travels through these systems.
This chapter will explore the essential components of these two systems and how we rely on them when running. We will refer back to this content in Part 2 when looking at training strategies. OK. Let’s jump in!
On the surface, the heart looks straightforward.
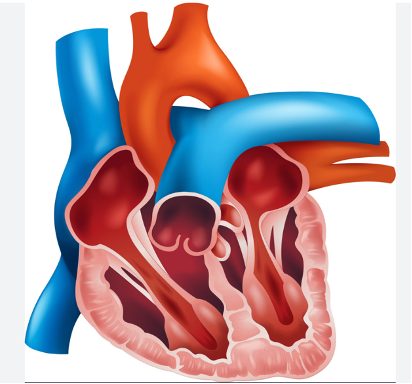
Which makes sense. If you have a piece of equipment that is always on, you don’t want hundreds of pieces that can fail. There are paths where the blood goes in, others where the blood goes out, and the muscles and valves that enable these actions.
Heart muscle is called cardiac muscle. A key difference from skeletal muscle, which moves our limbs, is that 25-35% of cardiac cells are composed of mitochondria, the energy producing components of cells.
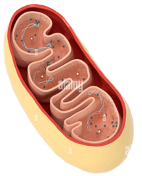
This compares to just 2% in skeletal muscle, reflecting the need for nonstop energy production to keep the heart beating, whereas demand on skeletal muscles is intermittent. Another key difference is cardiac cells are short, branched, and interconnected. This is important as cardiac cells need to rhythmically fire together. Also, the heart uniquely has pacemaker cells, which, as expected, set and maintain the heart rate.
The heart and aging
The heart is subject to aging processes. We noted in Chapter #3 that mitochondria are subject to free-radical oxidation to a greater degree than other cells. Since mitochondria are roughly 15 times more prevalent in cardiac muscle compared to skeletal muscle, free-radicals can disproportionally affect the heart — another good reason to take steps to minimize free-radical oxidation. These include a diet with fruits, vegetables, whole grains, low-processed foods, and staying hydrated.
Thickening and stiffening of heart valve flaps
Other age-related issues include the thickening and stiffening of heart valve flaps and the replacement of functioning cardiac cells with fibrotic scar tissue. Both of these things make the heart work harder and can eventually contribute to atrial fibrillation, an arrythmia increasingly seen in highly conditioned athletes who have years of hard training under their belt.
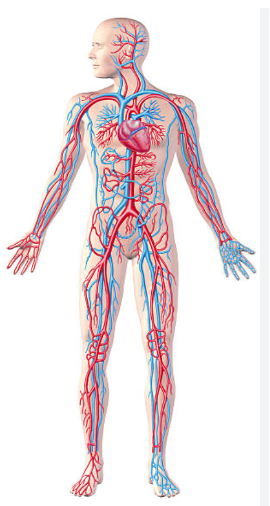
60,000 miles of blood vessels!
Blood leaves the heart loaded with oxygen, and then returns full of carbon dioxide. During that process it is subject to any constraints presented by our 60,000 miles of blood vessels.
Blood moves through a pressure gradient and if those miles of arteries, veins, or capillaries get clogged and/or stiffen, this makes the heart work harder to pump the same volume of blood. The result is increased blood pressure, for which age is often cited as a risk factor. But there is no secret about the things we have control over: good diet, non-smoking, quality sleep, and physical activity.
Cardiac Output: SV x HR
When looking at athletic performance, a key limiting factor is how much oxygen is delivered to our muscles. Since blood carries that oxygen, cardiac output is a vital measure. Cardiac output is the product of stroke volume and heart rate:
CO = SV x HR. Let’s look at these two components.
Stroke volume
Stroke volume is the amount of blood pumped with each beat. Typically, this is about half of the blood in the heart chamber. During exercise, our stroke volume is affected by how much our heart muscles can stretch to accept blood into the heart followed by the strength of the contraction of heart muscle that forces blood from the heart into the arteries. Studies have shown trained endurance athletes are able to increase stroke volume by up to 60% during exercise. Which makes sense: if all is working well, when we exert cardiac muscle stretches to accept more blood into the heart and then contracts harder to push it out. Also, a slower resting heart rate as a result of endurance training, is evidence the left ventricle is larger, providing a greater reservoir to receive blood.
Heart rate — the critical factor
However, the bulk of higher cardiac output during exercise is due to an increased heart rate. Here’s where age really comes into play. We grind up that steep hill, hoping our training has been sufficient to allow for a substantial increase in cardiac output. (Though we probably don’t think about it in those terms in the heat of the battle!) In any event and for various reasons, it’s tough sledding!
Heart rate formulas
So, what’s happening with our heart rate as we age? Various formulas for maximal HR are used. Here’s a table showing three methods. The generic 220-minus-age formula has been used for many decades and was developed with data drawn mostly from men. Based on newer data from 25,000 stress tests conducted with both men and women between ages 40 to 89 from 1993 to 2006, the American College of Cardiology (ACC) presented separate formulas for men and women. These new methods show women with lower maximal heart rates, perhaps due to hormonal differences. While continuing to show a significant reduction for both genders with age, the data suggests a greater retention of cardiac capacity than previously thought.
TraditionalBoth | ACCWomen’s | ACCMen | |
Age | 220 – Age | 208 – Age x.67 | 208 – Age x.70 |
40 | 180 | 173 | 180 |
60 | 160 | 160 | 166 |
80 | 140 | 146 | 152 |
% 40-Year Change | -22% | -16% | -16% |
Cardiac Reserve = Maximal Cardiac Output minus Resting Cardiac Output
There is one more thing to consider: our Cardiac Reserve. This is essentially what we draw on when putting the hammer down. When looking at resting cardiac output, we know conditioned athletes have lower resting heart rates. But as we noted, that training also tends to increase the volume of blood delivered per beat, even at rest.
Cardiac Ratio
It is the cardiac reserve we are trying build and maintain through our training. Taking it a step further, we are particularly interested in the cardiac ratio, a measure of athletic capacity, which is the Cardiac Reserve divided by our Resting Cardiac Output. For highly conditioned athletes, McArdle and Katch have shown this ratio can increase by a factor of six or even seven for elite athletes at top-end effort. From the above table, a well-trained 60-year old woman endurance runner with a resting heart rate of 45 might expect a ratio approximating McArdle’s and Katch’s findings.
Let’s put some numbers to this: The runner with a 160 maximal heart rate, a 45 resting heart rate, and a 60% increase in stroke volume would see a 5.7 times increase in cardiac ratio. Such a high cardiac ratio would certainly help when approaching the last mile of a 10K!
I suggest these findings provide good reason to do the things in training we know work: sufficient volume, regular speed work, a healthy diet, and restful sleep. It is during sleep that our bodies regenerate.
With this basic coverage of the cardiovascular system, we’ll move on to the respiratory system.
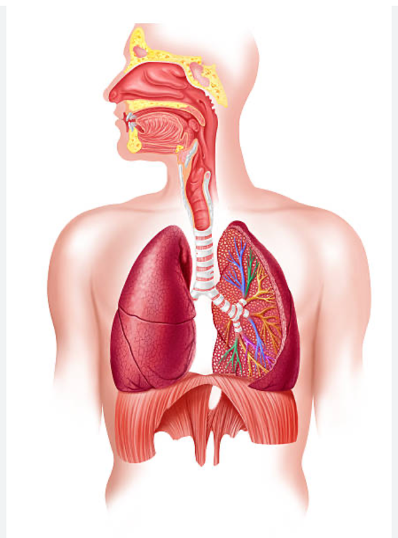
The respiratory system essentially has two functions:
One, breathing oxygen in and carbon dioxide out. This is largely mechanical. And two, diffusing oxygen from the lungs to the blood and then moving carbon dioxide back from to the blood to the lungs. This is a chemical process. A whole lot goes on in the body to enable this. Here, we will focus on the changes that happen when we run and how aging affects that.
Reaching our limits!
When running, our muscles call for more oxygen. We know very well the uncomfortable feeling of being out of breath! At some point, we top out – we simply can’t breathe harder or faster. The initial increase is due to neural impulses – we decide to start moving, which leads to a voluntary activation of muscles. This in turn activates the breathing apparatus. The cycle continues until we reach peak cardiac output as well as hitting two critical set points:
(1) Oxygen in; CO2 out. Capillaries in our lungs reach their maximum capacity to transfer oxygen in and carbon dioxide out;
(2) Muscular capacity to consume O2. Muscles reach their limit for consuming oxygen.
Increasing and maintaining these capacities is a primary objective of endurance training at any age.
Now, we look at the ventilation rate, which is the amount of air that flows in and out of our nose and mouth in a minute. At rest, this is around 6 liters; at peak exercise it may be 20-25 times that. This does not mean we are breathing 25 times faster. That would be hyperventilation, hardly a productive way to exercise. The increase, then, is a function of both our breathing rate, which may go up four times during intense exercise, and the tidal volume, the amount of air inspired with each breath, which may go up at much as six times.
Part of this increase is due to the elasticity of our lungs. This is called lung compliance. Regular exercise helps retain this compliance, whereas inactivity or disease degrade it. A related factor is thoracic compliance, a function of our diaphragm muscles and rib cage, which likewise retain elasticity with use.
A common measure of lung function is vital capacity, which describes the amount of air we can forcibly breathe in and then breathe out. As with all tissues, age results in an increased proportion of cellular cross-linkages (discussed in chapter 3) in our lungs, thoracic cavity, and chest wall. This in turn diminishes elasticity, meaning we work harder to accomplish the same output.
Air the limiting factor?
While the amount of oxygen available through the respiratory system may seem like the limiting factor in running performance, unless our lungs are compromised, we typically have sufficient air to breathe. Rather, it is the internal delivery of oxygen to our tissues and extraction of carbon dioxide that limits us. Our training is geared toward maintaining and increasing these delivery and extraction capacities.
I’ve kept this chapter brief. There is much to consider when looking at these vital systems and you may be inspired to dive deeper into them on your own. The main takeaways for our purposes are:
- The human highway, comprised or our cardiovascular and respiratory systems, is an intricate system. It gives us the means to go from here to there. Just as our road highways need regular care and maintenance, so too do these systems.
- As we age, there are physiological changes in our capacities (1) to deliver oxygen to our muscles, (2) to absorb this oxygen, and (3) to remove the carbon dioxide generated. We can be frustrated by this, or we can recognize it is happening and focus our training on those things that make the most difference. This includes sufficient training at an intense level.
- The rate of change for each senior runner varies significantly. To say, everyone’s VO2 max will decline 10% per decade does not honor the principle that we are experiments of one. Yet, whatever our baseline and genetic rate of change, we can train smart and retain as much function as possible.
The next chapter explores an equally complex group of systems: The nervous, endocrine, and immune systems. Please return for that discussion, sign up for updates if you have not already done so and send a link to fellow senior runners.